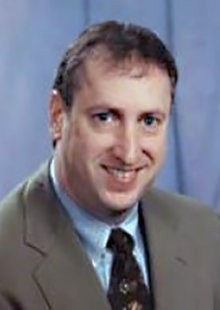
ABOUT
Dr. Speyer joined the MSE faculty in August, 1992 after serving on the faculty at the New York State College of Ceramics at Alfred University for six years. He has written one book (Thermal Analysis of Materials), with another one on the way, published over 125 refereed papers and has given over 150 technical presentations.
His present research group consists of seven graduate students and one Ph.D-level scientist. Dr. Speyer’s research has been funded by Navy, ARO, AFOSR, DARPA, Gas Research Institute, and private industry. He was previously the president of Innovative Thermal Systems, a thermoanalytical scientific instrument company, and is presently the President of Verco Materials, a start-up company which will manufacture boron carbide armor .
He teaches courses in Chemical Thermodynamics of Materials, Thermal and Transport Properties of Materials, and Ceramic Technology.
EDUCATION & AWARDS
- B.S. (1980)
- M.S. (1981)
- Ph.D. (1986), Ceramic Engineering, University of Illinois at Urbana-Champaign
RESEARCH INTERESTS
Boron Carbide Armor
Boron carbide is of great importance to the military for lightweight personal armor, and has saved countless American lives in both the Iraq and Afghanistan conflicts. The armor plates used for this application are hot-pressed, preferred by the fact that ballistic performance is strongly related to a close approach to theoretical density, and the belief that B4C, as other refractory covalent ceramics, does not sinter well.
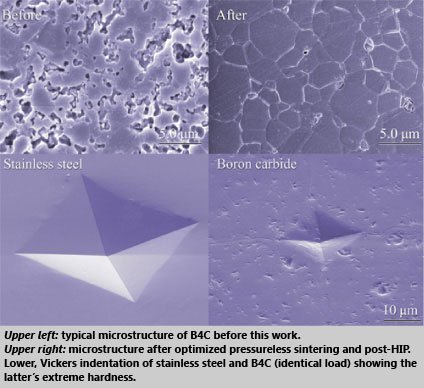
Research by Prof. Speyer and his students over the past five years have shown this belief to be false. Using a specially-built differential dilatometer capable of heating to well over 2500°C, in-situ measurements of contraction, CTE, weight loss, and particle size have permitted elucidation of the concurrent particle coarsening processes which attenuate the driving forces for sintering. This fundamental understanding permitted use of selected flowing atmospheres at specific temperatures, and a multi-step thermal schedule, which circumvents these coarsening processes, resulting in fired densities comparable to those obtained with hot pressing.
Beyond the economies associated with pressureless sintering, the great advantage of this development is the ability to cast and sinter dense parts of complex shape. The U.S. Army Soldier Systems Center in Natick MA, as well as the Army Research Slip cast B4C green body thigh protection plate. Laboratory in Aberdeen MD have shown a great interest in this technology for their next-generation body armor, which is of a more contoured shape that cannot be formed by gang-hot pressing, as well as helmets and other body-part protection systems. More recently, pressureless-sintered specimens have been post-hot
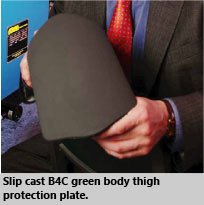
isostatically pressed to 100% of theoretical density, giving it a higher density and Vicker's hardness than commercially hot-pressed B4C, yet complex shapes can still be formed and retained. Slip casting and injection molding technologies have been developed in Dr. Speyer's laboratory to form B4C helmets and shin/thigh plates.
DARPA is funding Dr. Speyer's laboratory to develop pressureless sintering methods for nano-scale powders. [H. Lee, W. S. Hackenberger, and R. F. Speyer, J. Am. Ceram. Soc., 85 [8] 2131-2133 (2002), H. Lee and R. F. Speyer, J. Am. Ceram. Soc., 86 [9] 1468-1473 (2003), N. Cho, Z. Bao, and R. F. Speyer, J. Mat. Res., 20 [8] 2110 -16 (2005).]
Prior to the consolidation of the Ceramic, Metallurgical, and Polymer disciplines into Materials Science and Engineering, each undergraduate program entrusted classic texts to cover much of their curriculum—e.g. Kingery, Bowman, and Uhlmann's Introduction to Ceramics for Ceramists, Reed-Hill's Physical Metallurgy Principles for Metallurgists, and Rodriguiz's Principles of Polymer Systems for Polymer Scientists.
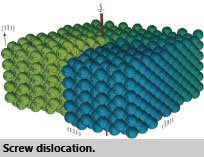
To date, there is no textbook which evenly covers the combined undergraduate discipline of Materials Engineering, beyond the introductory level (e.g. books by Callister and Shackleford, which were generally written with non-materials majors as their primary audience). Materials Engineering is written with the care and patience to be the book of our discipline. This book will be important in a number of respects. Many of our prominent textbooks are out of, or going out of print, and are not being
replaced by other books covering our core topics. Books that remain in print, by and large still follow the tracks of the three disciplines, imposing redundancy in presented concepts to MSE courses which follow them. With a merged curriculum, a variety of topics must be omitted to fit a four year program, which encouraged by the available textbooks, leads to choppy coverage. Materials Engineering carefully condenses and interrelates topics, so that from its efficient coverage, a breadth of topics remain well -treated as a logically-developing story.
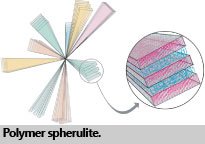
This, in turn, clears room in a curriculum for classes dealing with the cutting edge (nano and bio-materials), while a foundation based on the classical wisdom of our discipline is retained. These goals are facilitated by clear and vivid artwork, which imbue clarity with fewer words, decorating well-written chapters. The book would serve two purposes in an undergraduate curriculum. The first 5-6 chapters follow the perfection-to -imperfection
progression popularly used in introductory materials texts, but in greater depth. These chapters would cover fewer topics than in the non-major course, but in great enough detail that they would not need to be repeated in courses later in the undergraduate program, in turn facilitating course consolidation. Chapters 7 nucleationthrough 11 are individually long chapters, each of which cover the major content of individual courses in the undergraduate program.
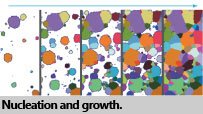
This book will thus decrease the number of textbooks imposed on student budgets, and provide a friendly, unified and interconnected treatment of these topics as students work their way through the curriculum.
It is expected that the book will be ready in about three years, and will have a significant impact on the Materials community.
Bridgman Crystal Growth
Dr. Speyer's group has designed and built two Bridgman single-crystal growth furnaces for TRS Ceramics for the fabrication of PMN single-crystal actuators. These actuators display ten times the displacement for a given voltage as
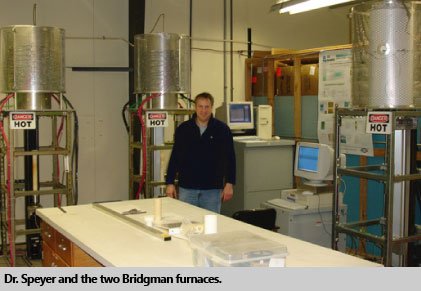
compared to polycrystalline ceramics of the same composition. The system has sixteen independently controlled heating zones, permitting the implementation of exotic temperature gradients along the crucible, which is lowered and rotated using high-precision stepper motors. Fifty seven thermocouples are used for furnace feedback control and monitoring, including two thermocouples on the rotating stand in direct crucible contact, connected through rotary mercury contacts. The system is designed to pull crystals either by mechanical lowering (over a period of two weeks) or through morphing of the temperature profile. The software developed by Dr. Speyer has permitted a wide variety of optimization experiments to be undertaken. Based on development with these systems, TRS has been able to zone-melt single -crystal actuators so that large boules of uniform composition and hence properties may be formed. Dr. Speyer’s group has since designed a new Bridgman furnace for TRS which has a 12 mm melt zone, which will further advance the perfection and yield of these single crystals.
Thermal Analysis of Materials Textbook
This text/reference book is in its second printing, and is an essential text for the new owners of thermoanalytical instrumentation. The book bases its treatment on elementary physical chemistry, heat transfer, materials properties, and device engineering. It stands apart from other books in the field since it develops a fundamental intuition into the nuances of such instrumentation, rather than an serving as an enumeration of literature citations. The book starts with the basic concepts of heat flow, temperature measurement, furnace design, feedback control logic and electronics. The longest, and most detailed chapter follows on differential thermal analysis. It dispels much confusion about differential thermal analysis versus differential scanning calorimetry, and details the experimental methodology required to generate reproducible transformation temperatures, as well as thermodynamic and kinetic data purged of instrumental effects. The manipulation of data chapter shows how programming languages can be used to numerically differentiate, integrate, etc., thermoanalytical (and other) data. Chapters on thermogravimetry and advanced applications show how thermoanalytical data can be fit to phenomenological models to deconvolute superimposed reactions, and measure phase equilibria in multicomponent systems. The dilatometry/interferometry chapter develops topics for the most industrially relevant thermoanalytical instruments, used for thermal expansion matching of various components of a high-temperature system. The pyrometry and thermal conductivity chapters detail the radiative properties of materials at very high temperatures, and how this can be exploited for contactless temperature measurement. This treatment also fills an important gap in undergraduate engineering education, in which a great majority of undergraduate students study heat transfer calculations, but are less informed on how to perform heat transfer measurements, e.g. determining thermal conductivity, thermal diffusivity, and radiant emittance. [R. F. Speyer, Thermal Analysis of Materials, Marcel Dekker, Inc., New York, 1994.]
Radiant Efficiency of Gas Radiant Emitters
The articles which comprise this work describe the development of a one-of-a-kind evaluation facility, which was used to elucidate the necessary features of high efficiency gas radiant burners. These burners act as gas light bulbs—a fuel air mixture ignites just-downstream of a porous ceramic or refractory metal membrane, convecting heat to the solid surface which in turn radiates to a load. Using a fused silica capillary on a computer-controlled mobile stage feeding a quadrapole
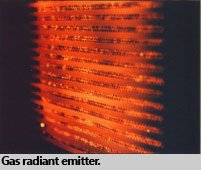
mass spectrometer, the flow rates and mixtures which encouraged flame liftoff could be clearly determined. Using a spectral radiometer and a numerical optimization routine, the temperatures and emittances of radiating surfaces could be accurately determined. By comparing the spectral emittance of CO2 combustion products and solid surfaces, the temperature differences between burner and exiting gas were determined, and used to explain the differences in efficiencies of a variety of commercial emitters. The role of flame support layers was divulged using specially-built burners with variable fraction closed areas. By comparing to heat transfer models, it was shown that these layers functioned to extract additional heat of combustion from exhaust gases, and increased efficiency up to the point where they excessively blocked direct radiation from the burner surface to the load. These papers displayed some excellent science, which at the same time has had direct and significant impact on the radiant burner industry, affecting emitter design and market share. [R. F. Speyer, W. Lin, and G. Agarwal, Experimental Heat Transfer, 8 [1] (1995), 9 213-245 (1996), 9 247-255 (1996).]
Rate Controlled Sintering
The concept of rate controlled sintering (RCS) was originally conceived by Palmour; furnace power is feedback controlled based on the sintering shrinkage rate of a powder compact. The significance of Dr. Speyer's work was in the development
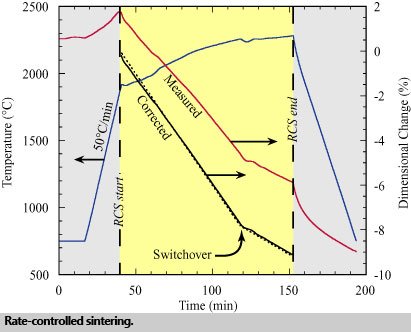
of instrumentation and software which purged experimental results of instrumental anomalies, so that the true merit of RCS could be proven. A purely radiant heating environment was used so that a much wider range of RCS schedules were possible. Dilation probe-induced specimen creep, residual sintering at the end of RCS, and thermocouple/ specimen temperature differences were eliminated by novel instrument design. Firing schedules to exacting sintered densities could be accomplished by in-situ software corrections for specimen dilation during sintering. Using pure ZnO as example, RCS was shown to form superior microstructures (minimum grain size and intragranular pore frequency) by following the most efficient thermal schedule required to achieve a desired level of densification—minimizing the time and thermal energy required for grain boundary movement. [G. Agarwal and R. F. Speyer, J. Mat. Res, 11 [3] 671-679 (1996).]
Three-Dimensional Rendering of Ternary Phase Equilibria
A software package was developed which generates a 3-dimensional ternary phase diagram representing liquidus, sub-liquidus, and solidus surfaces of the calcia-alumina-silica
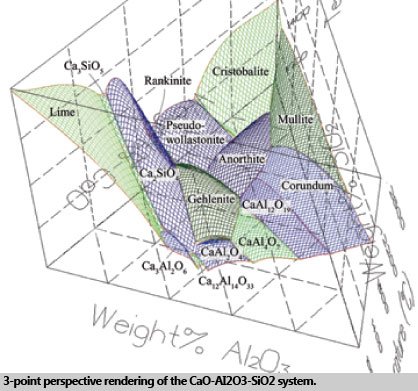
system, and allows user manipulation of the diagram to any selected viewpoint. A specific composition on the Gibbs triangle may be user selected, from which a rendering of the appropriate surfaces in the 3-dimensional object are rendered, as well as a user-interactive isoplethal study for that composition. A further feature is a movie-like continuously-changing rendering of isothermal sections with decreasing temperature. Both isoplethal studies and isothermal sections are user-interactive through the mouse position, generating compositions of phases and relative proportions at selected overall compositions and temperatures. The software functions as a powerful teaching tool in the visualization and understanding of ternary phase equilibria. When a description of the software was published in the American Ceramic Society Bulletin, over fifty requests for the software were immediately requested and provided. [R. F. Speyer, J. Phase Equilib., 17 [3] 186-195 (1996), Am. Ceram. Soc. Bull., 74 [11] 80-83 (1996)].
Deconvolution of Superimposed DTA/DSC Peaks
Deconvolution of superimposed x-ray diffraction peaks is an established and valuable procedure. In this paper,
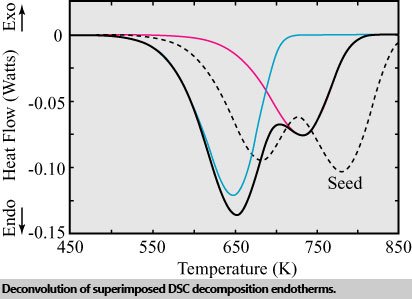
Dr. Speyer developed the mathematical models for fusion and decomposition reactions so that superimposed DTA/DSC endotherms could be deconvoluted using numerical optimization methods. In so doing, hidden onset temperatures, and the kinetic/thermodynamic parameters of parallel reactions can be elucidated using thermal analysis. [ R. F. Speyer, J. Mat. Res., 8 [3] 675-679 (1993).]
Fusion Paths of Complex Glass Batches with Reaction Accelerants
This work evaluated the reaction paths of five component glass batches (sand, soda ash, calcite, dolomite and feldspar) with reaction accelerants (e.g. NaCl). DTA traces of such batches have historically been of little use owing to their complexity. The merit of this work is the demonstrated methodology by which the individual reactions making up the trace could be elucidated using simultaneous thermal analysis (DTA and TG) of pairs, triples, etc. of batch constituents, x-ray diffraction, and deductive reasoning. The work showed the importance of dolomite in causing the first-formed liquid phase, and the local equilibria between the liquid phase and the sodium silicate phases surrounding remnant quartz. The glass industry has held this work in high regard, and is now an important procedure in their efforts to alter batch compositions to increase pull rates. [K. S. Hong and R. F. Speyer, J. Am. Ceram. Soc., 76 [3] 598-604 (1993), 76 [3] 605-608 (1993), M. E. Savard and R. F. Speyer, J. Am. Ceram. Soc., 76 [3] 671-677 (1993).]